My Research (Under construction)
The main focus of my research is to develop approaches and methods that can be utilized by terrestrial vegetation models for providing a parsimonious representation of the function and structure of plants and ecosystems. I use a combination of field measurements including eddy covariance, remote sensing, as well as physiological approaches. Learn more about my past and ongoing research projects below.
​
Sun-Induced chlorophyll Fluorescence (SIF) as an optical signal of water stress effects.
In 2004, as a Master student, I joined the Laboratory for Research Methods in Quantitative Remote Sensing (Quantalab, IAS-CSIC) led by PJ Zarco-Tejada to investigate the effects of water stress on leaf-level SIF measured with active sensors (i.e. pulse-amplitude-modulation (PAM) chlorophyll fluorometer) and its relationships with physiological parameters such as water potential, photosynthesis, and stomatal conductance. Although the theoretical foundations were already well established, there was very few evidences at that time that SIF could be measured using passive remote sensing methods at the crown level. Being encouraged by this challenge, we first demonstrated that water stress effects on SIF were detectable at the tree level from the in-filling effects of the O2-A band (Perez-Priego et al., 2005). Our results revealed as well that variations in field-measured SIF under different water stress conditions were also correlated with the photochemical reflectance index (PRI) at ecosystem scale (Suarez et al., 2008).
The effect of ChF on the apparent reflectance signal were only beginning to be explored and restricted to lab conditions. In this context, the study brought about significant methodological advances in remote sensing research, being one of the first comparing active and passive ChF measurements under field conditions and with natural light.
​
​
​
​
​
​
​
​
​
​
​
​
​
​
​
​
​
​
Figure. The plot above shows the relationship between field-measured leaf-level chlorophyll fluorescence (Ft) measured with PAM fluorometer and fluorescence in-filling extracted from crown reflectance through the amplitude of the 760-nm peak, calculated as R760.59-R759.5, on all trees under three stress levels. The lowest values of Ft and R760.59-R759.5 are associated with water stress (Perez-Priego et al., 2005).
​
Water Stress effects on Water-Use efficiency (PhD Thesis)
Improving plant water-use efficiency (WUE, the amount of CO2 uptake per unit of water loss) by regulated deficit irrigation (RDI) strategies is a fundamental research subject in agriculture of semiarid regions. The application of RDI has proven to save a significant amounts of water with moderate reductions in yield. However, whether this improvement is caused by an increased WUE is not clear due to the difficulty in upscaling leaf-level measurements to whole plant WUE. Yet, the physiological causes of an increased WUE by water stress are poorly understood at the whole-plant scale. Below some of the questions I faced in my PhD thesis.
​
-
Improving the representativeness of observations of water-use efficiency
The lack of correspondence among leaf gas exchange and landscape-scale eddy covariance measurements imposes a severe limitation in our understanding of plant physiological processes. To fill this gap, I designed a new canopy chamber for measuring CO2 and water vapor fluxes of individual trees of up to 40 m3 under different degrees of water stress. Note that one of the novelties is that the chamber excludes soil fluxes and only aboveground fluxes are measured. Several tests with xylem sap flow measurements demonstrated that the impacts induced by the chamber’s modification of the canopy environment on transpiration were minor (Pérez-Priego et al., 2010).
​
​
​
​
​
​
​
​
​
​
​
​
​
​
​
​
​
​
​
​
​
​
​
​
​
​
​
​
​
​
Figure. Schematic drawing of the chamber, showing the opening windows and other components (top). The pictures (bottom) show the performance of the chamber during a field campaign in an olive orchard measuring CO2 and water vapor fluxes from single trees together with sap flow measurements. The plot on the right hand side shows an example of a diurnal pattern of canopy photosynthesis (A) and transpiration (E) from single trees under different water irrigation management. The reduction in A and E by water stress is highlighted (Pérez-Priego et al., 2010).
-
Spatial and temporal implications of increased water-use efficiency by regulated deficit irrigation (RDI).
Previous studies had shown that leaf-level WUE observations at single points in time were not influenced by moderate water stress in olive trees. Our results disagreed with these earlier results, revealing that whole-plant WUE increased with stress (see Figure below). These results suggested that instantaneous leaf-WUE measurements do not necessarily correlate with whole-plant WUE and might fail in representing the whole plant behavior (Perez-Priego et al., 2010). Although controversial, these findings have been confirmed in other species (Medrano et al., 2012), which indicates that the gradient in leaf traits and environmental conditions within the canopy should be better characterized. Interestingly, my results showed that not only spatial but also temporal patterns caused the increased WUE by RDI. The results revealed that the effects of water deficit on plant-WUE were much higher at the daily and seasonal scales than instantaneous WUE due to different changes in transpiration compared to photosynthesis. WUE for longer time scales such as daily or seasonal WUE can be understood as a weighted mean of transpiration. Small shifts of the weighting can hence lead to significant differences for long-term WUEs. For example, RDI led to decreased transpiration during afternoons compared to full irrigation so that the weighting shifted slightly towards times with lesser VPD (and high photosynthesis) led to distinctly enhanced WUE for RDI compared to full irrigation (Villalobos et al., 2012). These measurements provided original conclusions about whole-plant physiological performances and plant behavior associated with water stress and pointed out the faultiness of assessing water saving strategies from single point in time leaf-level WUE measurements not considering spatiotemporal patterns. We provided hence significant advances in water optimization strategies widely applied in precision agriculture. In addition, these results allowed the calibration of a model coupling canopy conductance and photosynthesis. Model-data fusion revealed large seasonal variations in model parameters, suggesting that the observed changes of the physiology of olive trees in response to water stress should be accounted for in ecosystem models.
​
-
Seasonally varying plant organ composition determines temperature response of whole plant respiration.
​
I studied the respiration in photosynthetic and non-photosynthetic plant organs for assessing the carbon (C) economy of a tree or stand (Pérez-Priego et al., 2014). In this work, I showed for the first time that tree-level measurements of respiration in large olive trees and additional results to understand how, and to what degree, seasonally varying plant organ composition determines total respiration and its response to temperature. I demonstrated that the temperature response of whole-plant respiration varies in relation to plant organ composition such that a single temperature sensitivity for plant respiration is not warranted. I hence developed an improved model of ecosystem respiration by accounting for energy-requiring growth and maintenance processes among leaves, fruits, and woody parts ((Perez-Priego et al., 2014)).
​
Investigating subsoil CO2 transport processes: The role of subterranean ventilation (Postdoc at University of Granada, 2011-2013)
​
During my doctoral studies, I acquired experience and skills in combining methods at different functional integration scales. From this perspective, I started working in top-down approaches to better describe single processes from eddy covariance fluxes. By then, Prof A Kowalski’s research group had uncovered the existence of abiotic processes on carbonate rocks (subterranean ventilation fluxes, Vs) that uncouple atmospheric exchanges from concurrent biological processes (photosynthesis and respiration, Figure 6). These processes of subterranean C storage and ventilation were only beginning to be explored but were difficult to isolate by using only eddy covariance CO2 fluxes. After my PhD, I moved to the Atmospheric Physics Group (GFAT) of Prof Kowalski at the University of Granada to investigate the importance of transport processes by subterranean ventilation in driving observed CO2 fluxes.
Figure. Schematic of mechanisms and processes involved in net ecosystem CO2 exchanges (Fc) over an ecosystem with carbonate soil. In dry periods, subterranean ventilation fluxes (Vs) affecting daytime Fc dominate both concurrent biological processes: ecosystem respiration (Reco) and photosynthesis (Fgp). A negative subterranean storage of CO2 (Ss<0) by Vs represents destocking (left panel). Higher soil temperatures are denoted in red while lower temperatures appear in violet (Perez-Priego et al., 2013). The panel on the right shows an example of the daily time course of Fc (blue circles) and estimated subterranean ventilation (Vs; green triangles) for the dry period. An improved physiological interpretation of Fc in terms of photosynthesis and respiration is achieved after deducting the subterranean ventilation component (red circles). Vs was deducted using the proposed partitioning method by Perez-Priego et al. (2013) that characterizes biological fluxes in terms of evapotranspiration through their link to carbon assimilation by stomatal conductance.
​
​
​
​
​
​
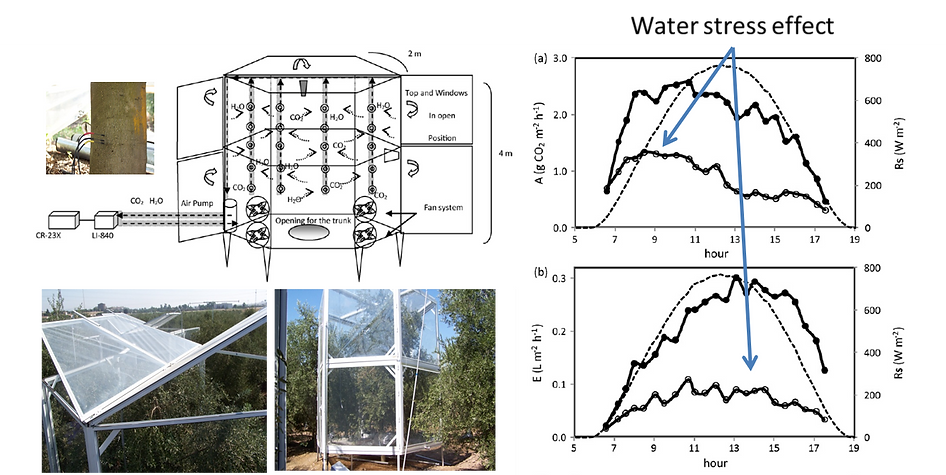

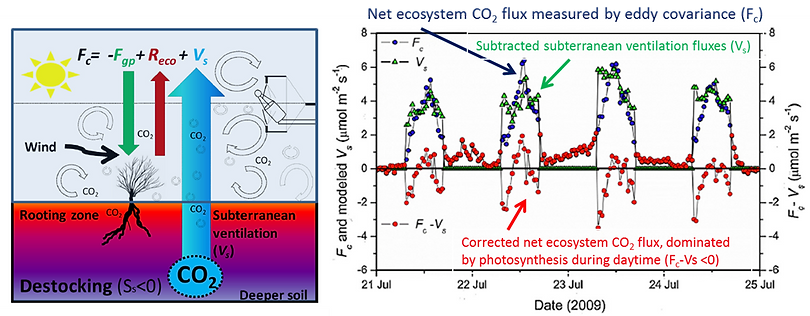